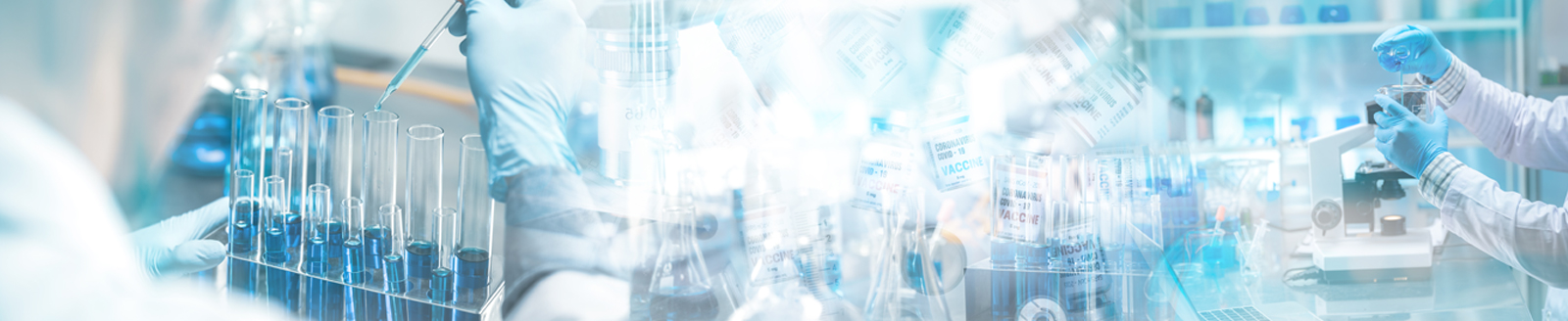
Science
Pioneering Gene Therapy for Inherited Retinal Diseases
Inherited Retinal Dystrophies (IRDs) are a group of genetic disorders causing progressive vision loss. These conditions arise from mutations in genes crucial for photoreceptor and retinal pigment epithelium (RPE) cell function. Retinitis Pigmentosa, the most prevalent form, affects an estimated 1.5 million people globally.
Our research pushes the boundaries of treatment for rare and challenging outer retinal layer disorders. While traditional therapies focus on symptom management, we target the root cause. For Retinitis Pigmentosa, especially cases with Rhodopsin (RHO) gene mutations, we’re pioneering innovative approaches using genome editing and HITI (Homology-Independent Targeted Integration) gene insertion techniques.
In collaboration with Hiroshima University, we’ve optimized highly specific Zinc Finger Nucleases (ZFNs), achieving editing efficiencies comparable to CRISPR-Cas9. Our collaboration with Kobe Eye Center Hospital is advancing precise and efficient delivery methods for these therapeutic agents. These efforts are paving the way for more effective and durable treatments, offering new hope to those affected by debilitating conditions.
Inherited Retinal Dystrophy (IRD)
Inherited Retinal Dystrophy (IRD) is an umbrella term for a group of genetic eye disorders that cause the progressive degeneration of photoreceptor cells. Among these, Retinitis Pigmentosa is the most common, affecting an estimated 1.5 million people worldwide. Genetic testing conducted by leading institutions, including Kobe City Eye Center Hospital, identified approximately 100 cases associated with these conditions. Most of these genes play crucial roles in maintaining the health and function of the RPE and photoreceptor cells. When mutations occur in these genes, it can trigger a cascade of degeneration, ultimately leading to photoreceptor cell death.
Unfortunately, there is currently no cure for Retinitis Pigmentosa. Treatment strategies mainly focus on managing symptoms and slowing the disease progression. These approaches include prescribing medications to enhance dark adaptation and improve blood flow, recommending vitamin supplements, and providing specialized sunglasses to shield eyes from harsh light. Although these methods can help patients cope with their symptoms, they do not address the underlying root cause of the condition.
Structure of the Eye and Retina
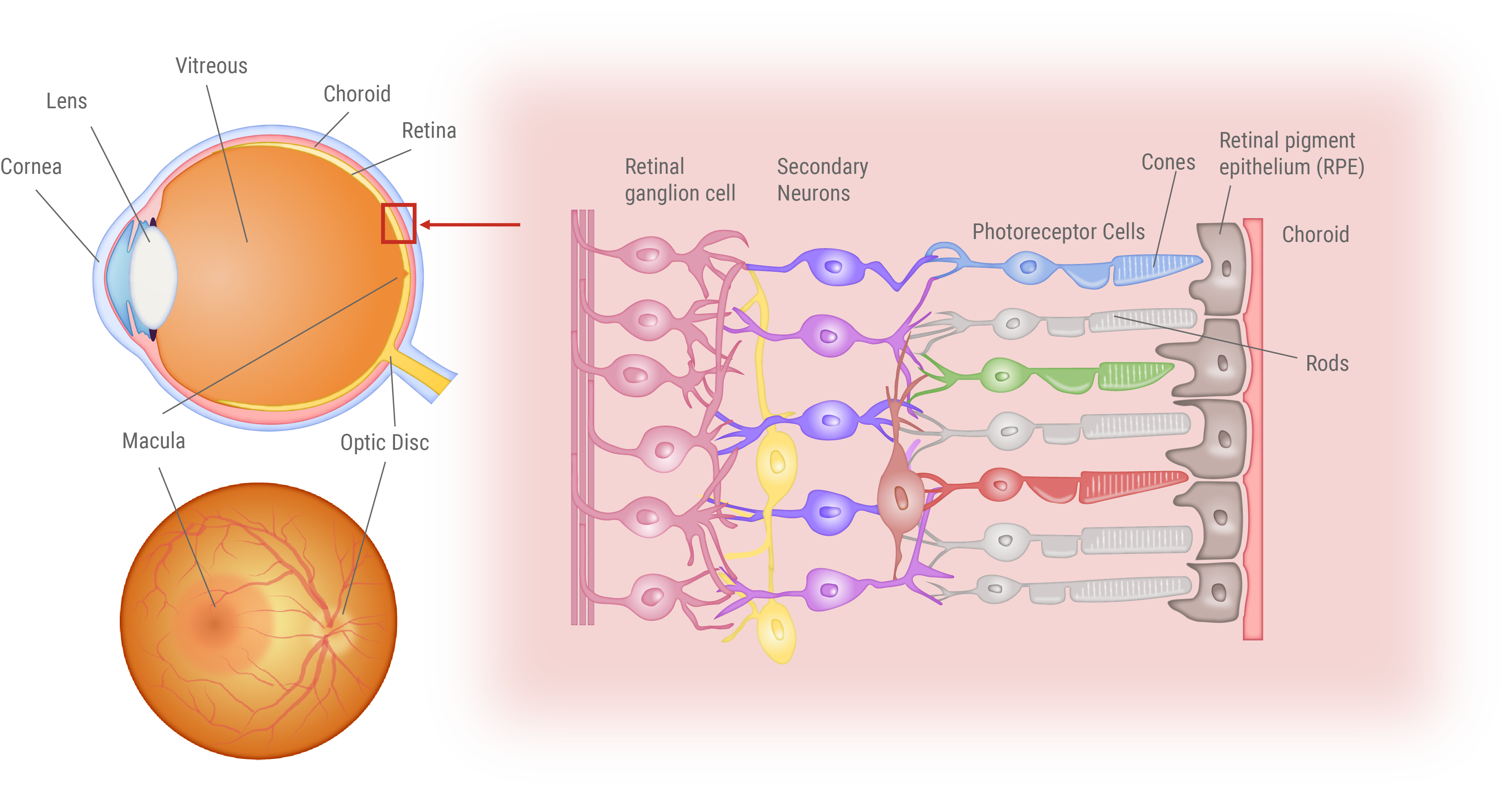
Visual Impairment in Retinitis Pigmentosa and Age-Related Macular Degeneration
This figure illustrates the distinct vision impairments experienced by patients with Retinitis Pigmentosa (RP) and Age-related Macular Degeneration (AMD). Patients endure a gradual loss of vision over time. For RP patients, the loss first occurs in the peripheral vision, progressively closing in on the center. In contrast, AMD patients first experience loss of central vision, making it challenging to focus on details.
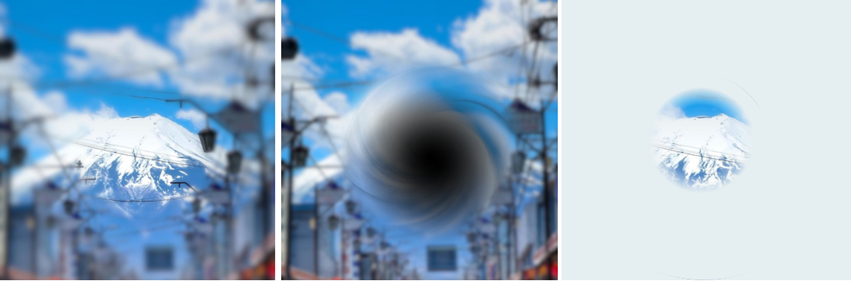
Gene-Targeted Therapeutics
Recent advances in science and technology have revolutionized medical treatment, expanding beyond traditional small-molecule drugs to include a wide array of therapies using polymers, nucleic acids, and even cells. This diverse range of innovative therapies and drugs is collectively known as “drug/medical modalities.” Among these, gene therapy has emerged as a groundbreaking approach that harnesses the power of genes (such as DNA or RNA) or targets a patient’s own genome.
Gene therapy often refers to treatments that directly address the root cause of genetic diseases. With recent advancements in genetic diagnosis and medical infrastructure, there is a growing interest in the potential of these therapies, especially for genetic disorders with known causative genes. While conventional treatments such as small molecules or antibodies have limitations, gene therapies promise more comprehensive and targeted effects.
Our research focuses on developing cutting-edge treatments for the most common form of Retinitis Pigmentosa, particularly for Rhodopsin (RHO) gene-associated mutations. By targeting the genetic root of the disease, we aim to offer more effective solutions than ever before.
Targeted gene therapeutics
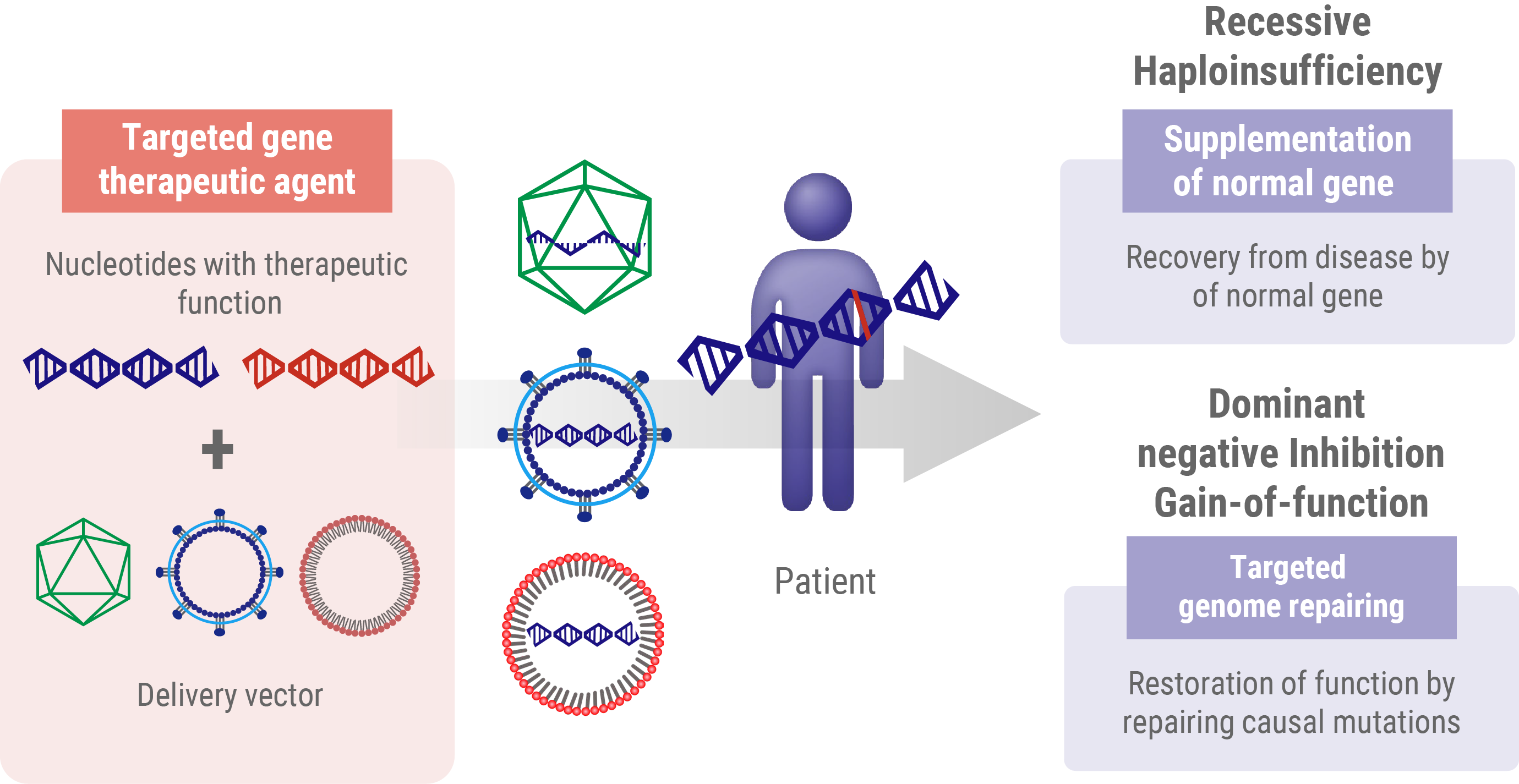
Gene Therapy for Recessive Mutations
In recessive mutations, the protein product does not work properly but does not interfere with normal protein function. Individuals with these recessive mutations only develop the disease when both alleles of a particular gene are mutated. In these cases, the go-to strategy in gene therapy is to introduce a working version of the gene from outside the body. This approach, known as gene supplementation, aims to fill the gap caused by loss-of-function gene products. Think of it like adding a fully functional spare part to a machine where both original parts are broken. By introducing this ‘spare part’ – the healthy gene – we’re aiming to restore the missing function and potentially slow or stop the progression of the disease.
Gene Therapy Strategies for Dominant Mutations
- Haploinsufficiency: In this scenario, a single functional allele is insufficient to maintain a normal physiological function. The therapeutic approach for haploinsufficiency is analogous to that used for recessive mutations, which involves the introduction of a wild-type gene to supplement inadequate protein production.
- Dominant-negative (or gain-of-function) mutations These mutations result in the production of an aberrant protein, which interferes with the function of the wild-type protein. Addressing dominant-negative mutations requires more sophisticated genome editing techniques to either correct or nullify the mutated allele.
Understanding these nuanced differences in mutation types and their effects is fundamental for developing effective, tailored gene therapy interventions for genetic disorders.
The Pathogenic Mechanisms of Rhodopsin Mutations in Photoreceptor Degeneration
Human Rhodopsin (RHO), a protein crucial for visual phototransduction, consists of 348 amino acids. Genetic analyses have identified more than 110 RHO-associated pathogenic mutations. Most of these mutations exhibit dominant-negative or gain-of-function characteristics, significantly altering protein function.
The functional consequences of these mutations are highly dependent on their location in the gene sequence. The observed effects include reduced photosensitivity, constitutive activation, and impaired intracellular trafficking of RHO protein. The severity of these inhibitory effects varies considerably among different mutations, necessitating precisely tailored therapeutic interventions through small-molecule compounds or other pharmacological agents.
Notably, RHO-associated pathologies can also arise from haploinsufficiency, in which a single functional allele is inadequate to maintain normal physiological function. The dual nature of RHO-related disorders presents a complex challenge for gene therapy approaches. Effective interventions must address both the correction of dominant-negative mutations and restoration of adequate wild-type RHO expression.
This multifaceted pathogenic mechanism underscores the need for comprehensive therapeutic strategies that can simultaneously mitigate the deleterious effects of mutant proteins and ensure sufficient levels of functional rhodopsins. This approach is critical for developing efficacious treatments for RHO-associated retinal degenerative disorders.
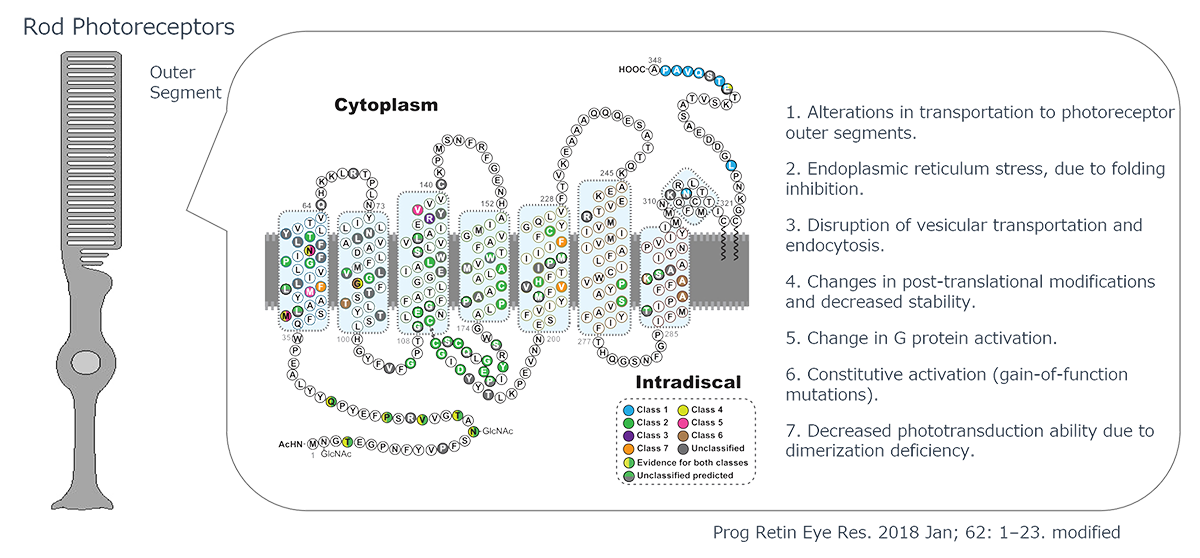
Genome editing-based therapy
Genome editing, a groundbreaking technique that allows us to rewrite the genetic code within cells, has tremendous potential for treating dominant-negative gene mutations. This innovative approach targets specific DNA sequences or mRNA transcripts in order to introduce precise genetic changes.
Our research is at the forefront of developing gene therapies with a strong emphasis on harnessing the power of genome editing. Through collaborative efforts with Hiroshima University, we have made significant strides in designing highly specific Zinc Finger Nucleases (ZFNs). These engineered ZFNs have shown remarkable efficiency in editing adult photoreceptor cells, rivaling the performance of the widely-used CRISPR-Cas9 system. We are also pushing the boundaries of gene therapy by developing treatments using Homology-Independent Targeted Integration (HITI) gene insertion techniques. This approach offers new possibilities for precise genetic modifications.
Genome Editing Methodologies
The predominant genome-editing strategy involves site-specific DNA cleavage at predetermined loci. These induced double-strand breaks (DSBs) activate two intrinsic cellular DNA repair pathways that can be exploited for gene insertion or targeted mutagenesis.
- Gene Insertion: This approach utilizes the homology-directed repair (HDR) mechanism. An exogenous DNA template, homologous to the target sequence but incorporating the desired modifications, is provided. During DSB repair, this template facilitates precise genomic alterations via recombination.
- Mutagenesis: This method leverages the non-homologous end-joining (NHEJ) pathway. NHEJ repairs DSBs by arbitrarily inserting or deleting nucleotides (InDels) at the break site. The stochastic nature of these InDels typically results in frameshift mutations or premature stop codons, which effectively disrupt the function of the target gene.
Genome editing via DNA repair machinery
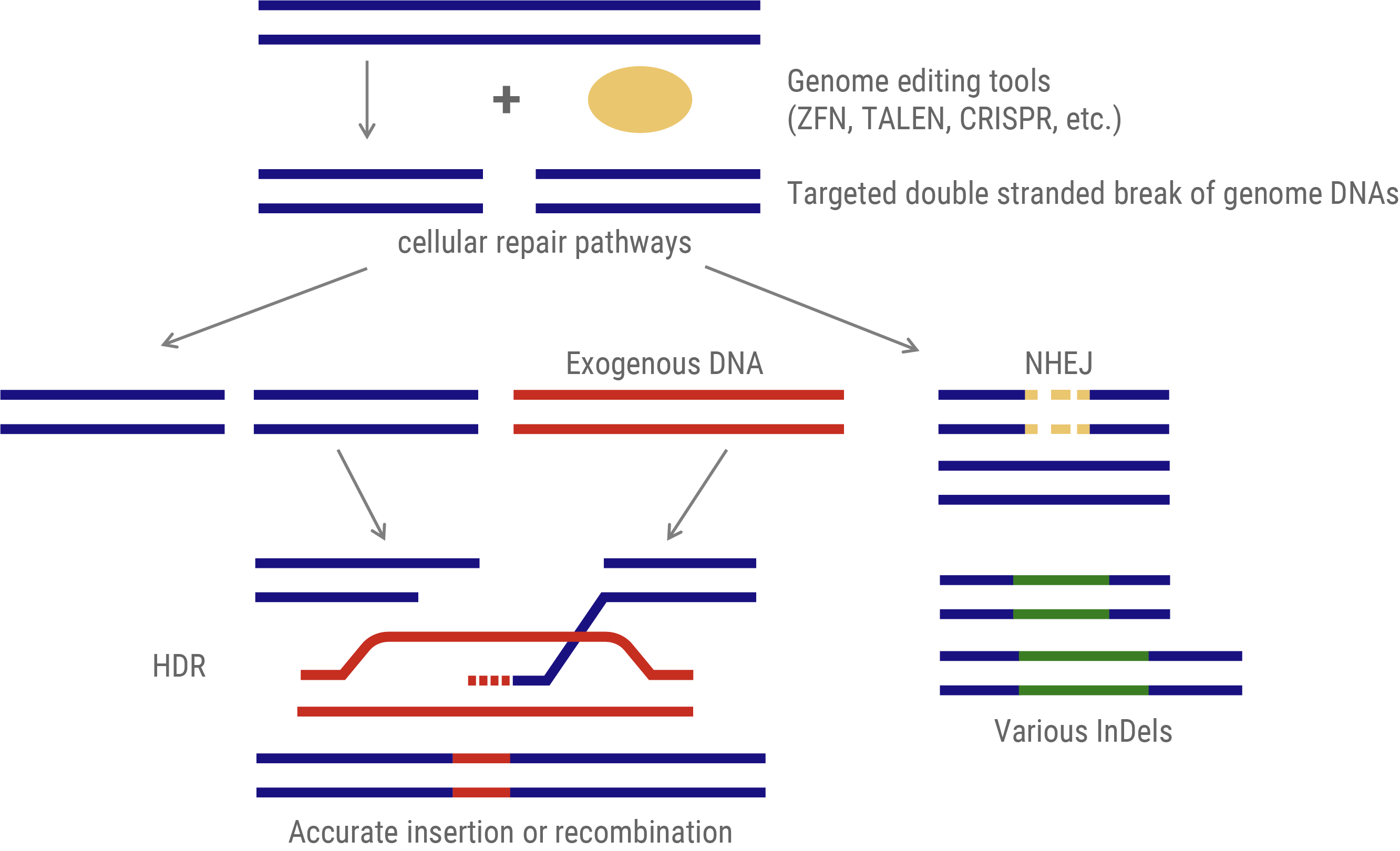
Genome Editing Tools: Evolutionary Progression
Enzymes employed in genome editing have evolved through three primary generations.
- First Generation – Zinc Finger Nucleases (ZFNs): These heterodimeric nucleases utilize DNA-binding motifs derived from zinc finger transcription factors. Each motif recognizes a trinucleotide sequence, allowing for the modular assembly of target-specific nucleases.
- Second Generation – Transcription Activator-Like Effector Nucleases (TALENs): Derived from bacterial DNA-binding proteins, TALENs offer enhanced specificity. Each TALE motif recognizes a single nucleotide, enabling the design of highly specific genome-editing tools with extended recognition sequences.
- Third Generation – Clustered Regularly Interspaced Short Palindromic Repeats (CRISPR): Based on prokaryotic adaptive immune systems, CRISPR technology comprises a Cas nuclease and single guide RNA (sgRNA). Unlike its predecessors, CRISPR employs RNA-DNA hybridization for target recognition, significantly simplifying target sequence design and accelerating genome editing research.
The advent of CRISPR has dramatically enhanced the accessibility and versatility of genome editing, catalyzing rapid advancements in the field of genetic engineering.
Highly efficient and Mutation-agnostic Gene Therapeutics to Treat Dominant Mutations
To address dominant mutations such as those affecting rhodopsin, a dual-action therapeutic approach is necessary. The gene therapy must simultaneously address the repair of the causative mutation inducing dominant inhibition and restore normal RHO gene expression. Given the presence of more than 110 potential mutation sites within the rhodopsin gene locus, a more efficient strategy involves the insertion or replacement of the full-length wild-type rhodopsin gene rather than developing mutation-specific therapies. This approach offers the potential to address all known mutations and accommodate newly discovered variants with a single therapeutic intervention.
Conventional approaches to introduce exogenous wild-type DNA sequences typically employ HDR-based genome editing. However, a significant challenge arises from the limited efficacy of HDR in post-mitotic cells, which constitute the majority of adult tissue cells, including the retinal neurons. To overcome this limitation, a homology-independent targeted integration (HITI) method has been developed. This innovative approach exploits the non-homologous end-joining (NHEJ) DNA repair pathway, which demonstrates high efficiency in non-dividing cells, to achieve highly efficient insertion of exogenous donor genes. Thus, HITI-mediated targeted gene therapeutics are ideal for targeting terminally differentiated adult cells such as retinal neurons.
By strategically positioning the wild-type sequence DNA immediately upstream of the start codon of the mutated gene locus, the expression of the normal gene is facilitated using the endogenous promoter, while concurrently suppressing the expression of the mutated allele. This elegant approach effectively addresses both the dominant-negative effects of the mutation and restores the wild-type RHO gene expression.
Our current research focuses on the development of HITI-based gene insertion therapeutics targeting the spectrum of rhodopsin mutations. This approach represents a significant advancement in the field of IRD therapeutics and offers the potential for a comprehensive treatment strategy for rhodopsin-associated retinal degeneration.
HITI-mediated gene-insertion therapeutics to treat RHO-associated patients
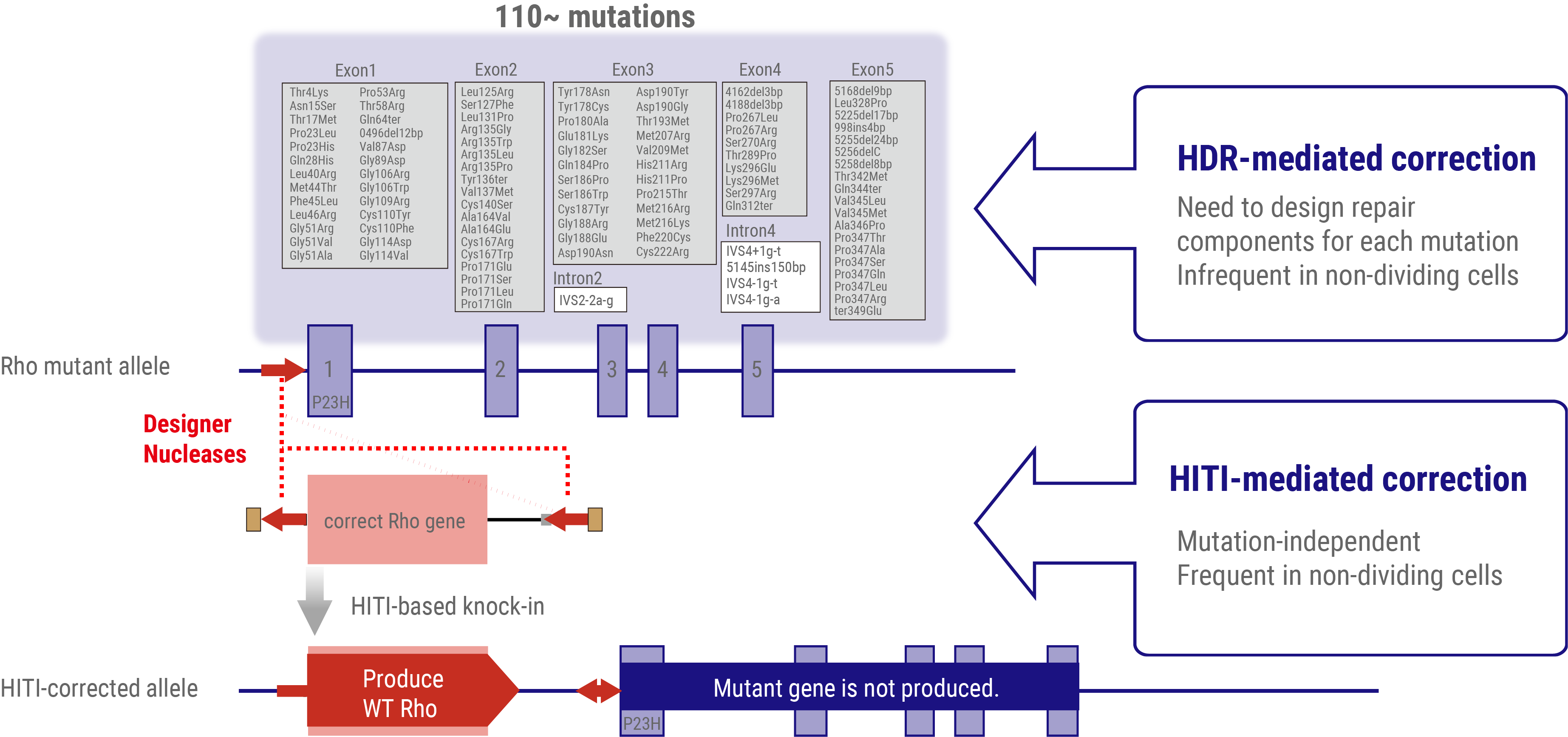
Zinc Finger Nuclease (ZFN)-mediated Genome Editing
Zinc Finger Nucleases (ZFNs) offer a distinct advantage in gene therapy applications owing to their relatively low molecular weight, which facilitates efficient packaging into viral vectors, such as adeno-associated viruses (AAV). However, the design of highly specific ZFNs for target sequences has traditionally been complex and time-consuming. Through collaborative research with Hiroshima University, we have developed a high-throughput methodology for generating highly specific ZFNs. Utilizing Hiroshima University’s proprietary FirmCut nuclease ND1, we established a ZFN development technology that achieves cleavage efficiencies comparable to those of CRISPR-Cas9 systems in terminally differentiated retinal cells.
Several critical factors must be optimized in the development of therapeutic agents employing ZFN-driven HITI-mediated gene insertion. These include the design of constructs to maximize gene functionality, selection of appropriate vectors for gene delivery, and refinement of the insertion techniques. Our research leverages insights from functional genomics and stem cell biology to inform the design of constructs with optimized gene functionality.
In collaboration with Synplogen Co., Ltd., Kobe, Japan, we pioneered the development of high-quality AAV vectors. Furthermore, our partnership with Kobe Eye Center Hospital is focused on advancing our understanding and methodology of safe and accurate gene delivery operations in the context of retinal tissue.
Novel genome editing tool with ZF (zinc finger) and FirmCut nuclease
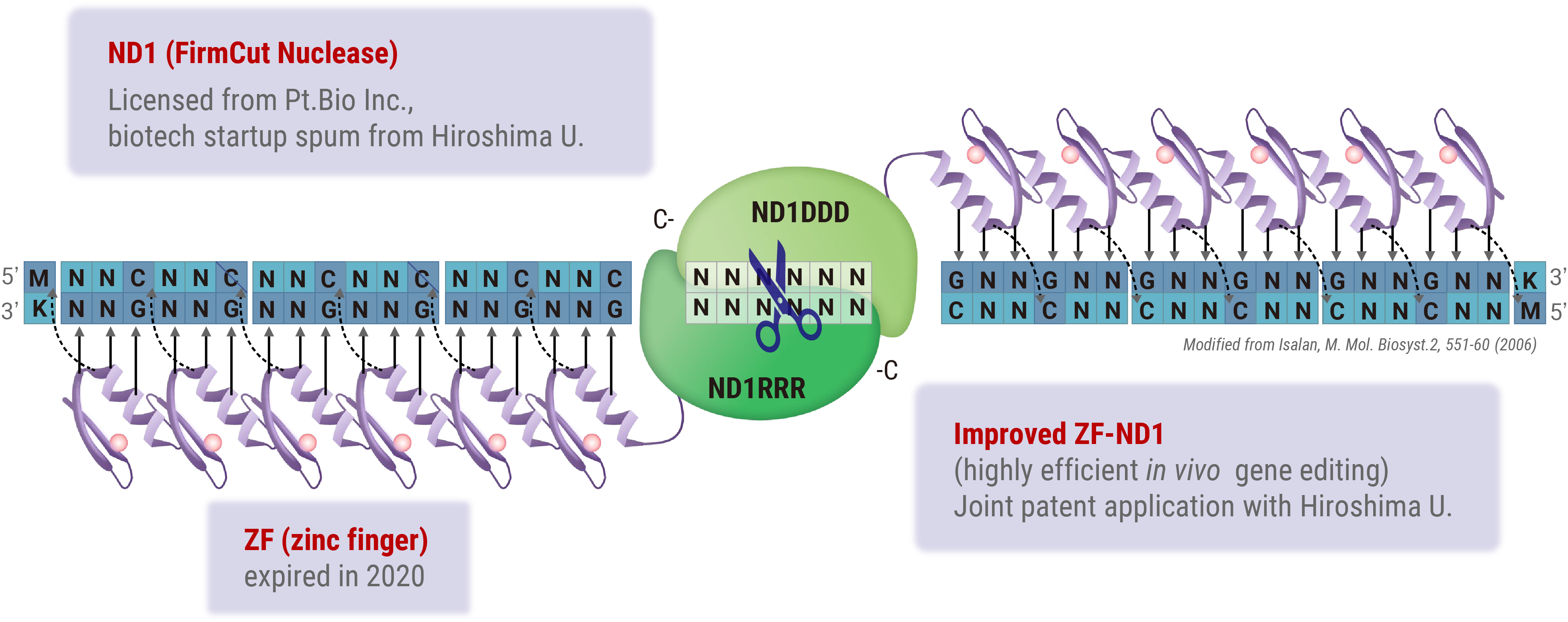